When The Controller Receives A Signal Below The Set Point, It Activates The:
12.09.2019 admin
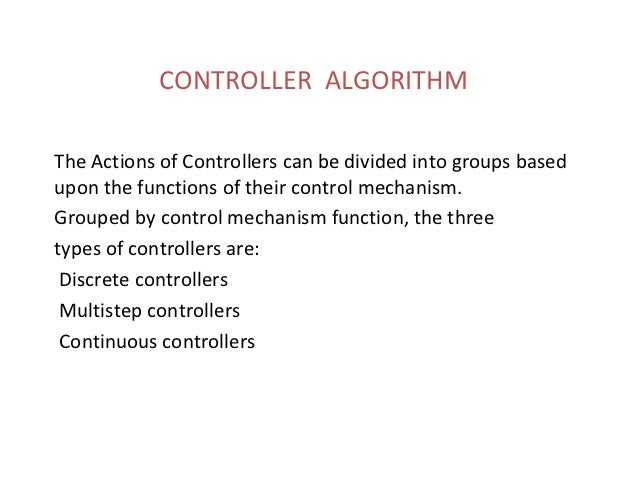
doi: 10.1152/advan.00107.2015
PMID: 26628646
In biology, homeostasis is the state of steady internal physical and chemical conditions. All homeostatic control mechanisms have at least three interdependent. When the signal is received and acted on, negative feedback is provided to. The temperature regulator's set point is reset during infections to produce a fever. This is why systems able to connect and control multiple thermostats have been developed. The system algorithms can analyze the information received by sensors at the. As the room or return air temperature falls below the set point 72°F. Results in an error signal that evokes the activation of effector responses (i.e.,.
This article has been cited by other articles in PMC.
Abstract
Homeostasis is a core concept necessary for understanding the many regulatory mechanisms in physiology. Claude Bernard originally proposed the concept of the constancy of the “milieu interieur,” but his discussion was rather abstract. Walter Cannon introduced the term “homeostasis” and expanded Bernard's notion of “constancy” of the internal environment in an explicit and concrete way. In the 1960s, homeostatic regulatory mechanisms in physiology began to be described as discrete processes following the application of engineering control system analysis to physiological systems. Unfortunately, many undergraduate texts continue to highlight abstract aspects of the concept rather than emphasizing a general model that can be specifically and comprehensively applied to all homeostatic mechanisms. As a result, students and instructors alike often fail to develop a clear, concise model with which to think about such systems. In this article, we present a standard model for homeostatic mechanisms to be used at the undergraduate level. We discuss common sources of confusion (“sticky points”) that arise from inconsistencies in vocabulary and illustrations found in popular undergraduate texts. Finally, we propose a simplified model and vocabulary set for helping undergraduate students build effective mental models of homeostatic regulation in physiological systems.
Keywords: homeostasis, negative feedback, regulation, core concepts
in 2007, a group of 21 biologists from a wide range of disciplines agreed that “homeostasis” was one of eight core concepts in biology (). Two years later, the American Association of Medical Colleges and Howard Hughes Medical Institute in its report (1) on the scientific foundations for future physicians similarly identified the ability to apply knowledge about “homeostasis” as one of the core competencies (competency M1).
From our perspective as physiologists, it is clear that homeostasis is a core concept of our discipline. When we asked physiology instructors from a broad range of educational institutions what they thought the “big ideas” (concepts) of physiology were, we found that they too identified “homeostasis” and “cell membranes” as the two most important big ideas in physiology (). In a subsequent survey (), physiology instructors ranked homeostasis as one of the core concepts critical to understanding physiology.
If, as these surveys indicate, the concept of homeostasis is central to understanding physiological mechanisms, one would expect that instructors and textbooks would present a consistent model of the concept. However, an examination of 11 commonly used undergraduate physiology and biology textbooks revealed that this is not necessarily the case (17). Explanations of the concept of homeostasis and subsequent references to the concept suffer from a number of shortcomings. Although these texts define some terms related to homeostatic regulatory systems, many authors do not use these terms consistently. Moreover, they do not always use consistent visual representations of the concept. In addition, the explanation of the concept often conflicts with the current understanding of homeostatic regulatory mechanisms. These limitations of textbooks most likely carry over to classroom instruction, thereby weakening the power of the concept as a unifying idea for understanding physiology.
The goals of this article are to develop a correct description and visual representation of a general homeostatic mechanism that can serve as a learning tool for faculty members and students. We will limit our discussion to homeostatic mechanisms found in organismal systems that maintain a constant extracellular compartment and will not consider other types of homeostasis. Although this tool can be useful at any academic level, our primary focus is its application at the undergraduate level when students are first introduced to the concept. We will also briefly discuss the history of the concept and then address the “sticky points” that may lead to confusion for faculty members and students alike when attempting to apply the concept to mammalian, organismal physiology. We conclude with suggestions for improving instruction on homeostasis and its applications.
History of the Concept of Homeostasis
Claude Bernard asserted that complex organisms are able to maintain their internal environment [extracellular fluid (ECF)] fairly constant in the face of challenges from the external world (). He went on to say that “a free and independent existence is possible only because of the stability of the internal milieu” (3). Walter Cannon coined the term “homeostasis” with the intent of providing a term that would convey the general idea proposed some 50 yr earlier by Bernard (). Cannon's view focused on maintaining a steady state within an organism regardless of whether the mechanisms involved were passive (e.g., water movement between capillaries and the interstitium reflecting a balance between hydrostatic and osmotic forces) or active (e.g., storage and release of intracellular glucose) (6). While we recognize the validity of both passive and active mechanisms of homeostasis, our consideration will focus exclusively on the active regulatory processes involved in maintaining homeostasis.
Early physiology textbooks reflected this broad definition by briefly mentioning Bernard's concept of the constancy of the internal milieu, but the term “homeostasis” was not used in discussions of specific regulatory mechanisms (9, 11, 4).
This situation began to change in the mid-1960s, when a branch of biomedical engineering emerged that focused on applying engineering control systems analysis to physiological systems (18, 19, 2, 20). Arthur Guyton was the first major physiology textbook author to include a control systems theory approach in his textbook, and his book included detailed attention to the body's many regulatory mechanisms (10). Hence, Guyton introduced many students to the concept of homeostasis as an active regulatory mechanism that tended to minimize disturbances to the internal environment.
Engineering control systems theory describes a variety of other mechanisms to maintain the stability of a system. Although many of these mechanisms may be found in biological systems (), not all of them are components of homeostatic mechanisms. For instance, the ballistic system used by the nervous system for throwing a ball simply calculates in advance the pattern of commands needed to achieve some particular outcome based on previous experience (). Here, there is no element involved that regulates the internal environment.
Homeostatic mechanisms originated to keep a regulated variable in the internal environment within a range of values compatible with life and, as has been more recently suggested, to reduce noise during information transfer in physiological systems (). To emphasize the stabilizing process, we distinguish between a “regulated (sensed) variable” and a “nonregulated (controlled) variable” (5, ). A regulated (sensed) variable is one for which a sensor exists within the system and that is kept within a limited range by physiological mechanisms (5). For example, blood pressure and body temperature are sensed variables. Baroreceptors and thermoreceptors exist within the system and provide the value of the pressure or temperature to the regulatory mechanism. We call variables that can be changed by the system, but for which sensors do not exist within the system, nonregulated (controlled) variables. Nonregulated variables are manipulated or modulated to achieve regulation of the variable being held constant. For example, heart rate can be changed by the autonomic nervous system to regulate blood pressure, but there are no sensors in the system that measure heart rate directly. Hence, heart rate is a nonregulated variable.
A simple model illustrating the fundamental engineering control system concepts relevant to homeostatic regulatory mechanisms is shown in Fig. 1.
Diagram of a generic homeostatic regulatory system. If the value of the regulated variable is disturbed, this system functions to restore it toward its set point value and, hence, is also referred to as a negative feedback system.
This model, some version of which appears in many current physiology texts, includes the following five critical components that a regulatory system must contain to maintain homeostasis:
1. It must contain a sensor that measures the value of the regulated variable.
2. It must contain a mechanism for establishing the “normal range” of values for the regulated variable. In the model shown in Fig. 1, this mechanism is represented by the “set point,” although this term is not meant to imply that this normal range is actually a “point” or that it has a fixed value. In the next section, we further discuss the notion of a set point.
3. It must contain an “error detector” that compares the signal being transmitted by the sensor (representing the actual value of the regulated variable) with the set point. The result of this comparison is an error signal that is interpreted by the controller.
4. The controller interprets the error signal and determines the value of the outputs of the effectors.
5. The effectors are those elements that determine the value of the regulated variable.
Such a system operates in way that causes any change to the regulated variable, a disturbance, to be countered by a change in the effector output to restore the regulated variable toward its set point value. Systems that behave in this way are said to be negative feedback systems.
While the model shown in Fig. 1 is a relatively simple one, there is a great deal of information that can be packed into each of the boxes that constitute the model. Homeostasis can also be described as a hierarchically arranged set of statements, a conceptual framework, that contains whatever breath and depth of information is appropriate for a particular set of students in a course. We have developed and described such an “unpacking” of the core concept of homeostasis (12, 13). The model and the conceptual framework provide students with different tools for thinking about homeostasis.
Topics That Cause Confusion for Students and Instructors: Sticky Points
A sticky point is any conceptual difficulty that makes one's mental model of any phenomenon inaccurate and, hence, less useful. There are a number of factors that contribute to the generation of sticky points for both instructors and students:
The phenomenon in question is a complex one.
There are aspects of the phenomenon that are counterintuitive.
The language or terminology used to describe the phenomenon or concept is inconsistent.
The discipline's understanding of the phenomenon is uncertain or incomplete.
In this section, we will describe some sticky points about homeostatic regulatory mechanisms that we have uncovered as we have interacted with instructors and students about their understanding of homeostasis. We will address these sticky points in the form of a series of questions and answers.
What environment is regulated by organismal homeostasis?
Organismal homeostasis, as originally defined by Cannon (6), refers to physiological mechanisms that maintain relatively constant the variables related to the internal milieu of the organism. This includes variables related to the entire ECF compartment or to its subcompartments (e.g., the plasma). We will not be discussing intracellular homeostatic mechanisms.
Are all negative feedback systems homeostatic?
Although negative feedback is an essential element of homeostatic regulatory mechanisms, the presence of negative feedback in a system does not mean that the system is homeostatic in function. Negative feedback exists in many systems that do not involve homeostatic regulation. For example, negative feedback plays a role in the muscle stretch reflex, but this reflex is not involved with maintaining the constancy of the internal environment. In other cases, the presence of negative feedback may minimize oscillation of a variable, even though that variable itself is not maintained relatively constant (i.e., it is not a regulated variable). Control of the blood levels of cortisol is an example of the oscillating damping effects of negative feedback (see further discussion below).
Can other types of control mechanisms (e.g., feedforward) maintain homeostasis?
Feedforward or anticipatory control mechanisms permit the body to predict a change in the physiology of the organism and initiate a response that can reduce the movement of a regulated variable out of its normal range (, ). Thus, feedforward mechanisms may help minimize the effects of a perturbation and can help maintain homeostasis. For example, anticipatory increases in breathing frequency will reduce the time course of the response to exercise-induced hypoxia. Because of this, attempts have been made to broaden the definition of homeostasis to include a range of anticipatory mechanisms ().
However, we have decided to limit our generic model of a homeostatic regulatory system (Fig. 1) to one that illustrates negative feedback and demonstrates the minimization of an error signal. We have done this because our model is intended to help faculty members teach and students learn the core concept of homeostasis in introductory physiology (12, 13). There are additional complex features found in feedback systems that are not included here because our intention is to first help students make sense of the foundational concept of homeostatic regulation. As situations are encountered where this basic model is no longer adequate to predict system behavior (, ), additional elements like feedforward mechanisms can be added to the model.
What is a set point?
Understanding the concept of a set point is central to understanding the function of a homeostatic mechanism. The set point in an engineering control system is easily defined and understood; it is the value of the regulated variable that the designer or operator of the system wants as the output of the system. The cruise control mechanism in an automobile is an example of a system with an easy to understand set point. The driver determines the desired speed for the car (the set point). The regulatory mechanism uses available effectors (the throttle actuators) and a negative feedback system to hold the speed constant in the face of changes in terrain and wind conditions. In such a system, we can envision an electronic circuit located in the engine control module that compares the actual ground speed with the set speed programmed by the driver and uses the error signal to control the throttle actuator appropriately.
In physiological systems, the set point is conceptually similar. However, one source of difficulty is that, in most cases, we do not know the molecular or cellular mechanisms that generate a signal of a particular magnitude. What is clear is that certain physiological systems behave as though there is a set point signal that is used to regulate a physiological variable ().
Another challenge to our understanding of set points arises from the fact that set points are clearly changeable, either physiologically or as the result of a pathological change in the system (). The mechanisms that cause variations in a set point can operate temporarily, permanently, or cyclically. Physiologically, this can occur as a result of discrete physiological phenomena (e.g., fever), the operation of hierarchical homeostats (e.g., regulation of ECF Pco2) (see Ref. ), or through the influence of biological clocks (e.g., circadian or diurnal rhythms of body temperature). The observation that set points can be changed adds complexity to our understanding of homeostatic regulation and can lead to confusion about whether the measured change in a regulated variable results from a change in the physiological stimulus or from a changing set point (). In these cases, it is important to make such distinctions between a change in the stimulus and the modulation of the set point to arrive at an accurate picture of how a particular homeostatically regulated system operates.
Do homeostatic mechanisms operate like an on/off switch?
Control signals are ALWAYS present, and they continuously determine the output of the effectors. Changes in the control signals alter effector outputs and therefore change the regulated variable. The amplitude of these control signals vary when there is an error signal (i.e., when the regulated variable is not the same as the set point). Thus, homeostatic regulation is a constant, continuous process and does not ordinarily operate as an on/off switch that results in an all-or-none response.
What is the difference between an effector and a physiological response?
Textbook diagrams and narratives can blur the distinction between the effector and a response generated by the effector, making it difficult for students to build a correct mental model. This problem can occur if, when a visual representation of a homeostatic mechanism is presented (see Fig. 1), a physiological response is placed in the same “concept” box as the effector. For example, “increased secretion by sweat glands” and “vasodilation of blood vessels in the skin” might be identified as effectors in the control system for thermoregulation. However, only “sweat glands” and “blood vessels” are effectors, whereas “increased secretion” and “vasodilation” are the responses of the effectors. Comprehensive understanding of homeostatic mechanisms requires that we, and students, make clear distinctions between effectors and responses. The term “effector” should only be applied to a physical entity such as a cell, tissue, or organ, whereas responses such as secretion and vasodilation are actions, not physical entities.
Students may also be confused if only the change in the regulated variable is thought of as being the response of the effector. The change in the regulated variable is typically a consequence of changes in function caused by effectors that determine the value of the regulated variable. By applying the term “response” to only the change in the regulated variable, the intermediary steps between the action of the effector and the change in the regulated variable are not acknowledged explicitly. Under these circumstances, it would reasonable for students to conclude that the intermediary steps are, in some way, aspects of the effector rather than the effect of actions of the effectors. This practice may also reflect a lack of understanding of the difference between the regulated variable, e.g., body temperature, and all of the nonregulated variables that are modified (e.g., arteriole diameter and rate of sweat production) in the steps between the action of the effector and the change in the regulated variable.
What does “relatively constant over time” mean?
In the above sections, we emphasized that homeostatic mechanisms operate to keep a regulated variable in the internal environment “relatively constant.” This is a common phrase used to describe what normally happens to the value of the regulated variable over time. A potential sticky point arises from the use of this phrase. How much change can occur to a regulated variable that is held relatively constant? Three points of clarification need to be made. By saying relatively constant, we mean that:
1. Regulated variables are held within a narrower range of values than if they were not regulated.
2. The regulated value is maintained within a range that is consistent with the viability of the organism.
3. There are differences in the range of values permitted for different regulated variables.
The second point is key to understanding the range over which regulated variables can change; homeostatic mechanisms operate to prevent a potentially lethal change in the internal environment. Indeed, as it is often used, relatively constant essentially serves as a surrogate phrase for within the range compatible with an organism's viability. For some regulated variables, the range is quite narrow (e.g., extracellular H+ concentration or extracellular osmolarity). For other variables, the range can be broad under some circumstances (e.g., blood glucose concentration during the fed state) and narrow in other situations (e.g., blood glucose during the fasting state). The factors that contribute to the normal range or, in our model, the set point, of a particular variable are undoubtedly complex and, in most cases, have not been elucidated.
What physiological variables are homeostatically regulated?
To identify specific variables that may be homeostatically regulated, the five critical components illustrated in the model shown in Fig. 1 must be present. That is, a regulatory system for that variable must exist that contains the five critical components described in Fig. 1. Based on this test, we have generated a partial list of the physiological variables that are homeostatically regulated (Table 1). The list of widely recognized and clearly established regulated variables in humans includes a number of inorganic ions (e.g., H+, Ca2+, K+, and Na+), blood-borne nutrients (e.g., glucose), blood pressure, blood volume, blood osmolarity, and core body temperature.
Table 1.
Homeostatically regulated variables typically found in undergraduate human physiology textbooks
Regulated Variable | Normal Range or Value | Sensor (Location If Known) | Control Center (Location) | Effectors | Effector Response |
---|---|---|---|---|---|
Arterial Po2 | 75–100 mmHg | Chemosensors (carotid bodies and aortic body) | Brain stem | Diaphragm and respiratory muscles | Change breathing frequency and tidal volume |
Arterial Pco2 | 34–45 mmHg | Chemosensors (carotid bodies, aortic body, and the medulla) | Brain stem | Diaphragm and respiratory muscles | Change breathing frequency and tidal volume |
K+ concentration | 3.5–5.0 meq/l | Chemosensors (adrenal cortex) | Adrenal cortex | Kidneys | Alter reabsorption/secretion of K+ |
Ca2+ concentration | 4.3–5.3 meq/l (ionized) | Chemosensors (parathyroid gland) | Parathyroid gland | Bone, kidney, and intestine | Alter reabsorption of Ca2+, alter resorption/building of bone, and alter absorption of Ca2+ |
H+ concentration (pH) | 35–45 nM (pH 7.35–7.45) | Chemosensors (carotid bodies, aortic body, and floor of the fourth ventricle) | Brain stem | Diaphragm and respiratory muscles | Change breathing frequency and tidal volume and change secretion/reabsorption of H+/bicarbonate ions |
Chemosensors (kidney) | Kidney | Kidney | |||
Blood glucose concentration | 70–110 mg/dl | Fed state: chemosensors (pancreas) | Pancreas | Liver, adipose tissue, and skeletal muscle | Alter storage/metabolism/release of glucose and its related compounds |
Fasting state: chemosensors (hypothalamus, pancreas) | Hypothalamus | ||||
Core body temperature | 98.6°F | Thermosensors (hypothalamus, skin) | Hypothalamus | Blood vessels and sweat glands in the skin as well as skeletal muscles | Change peripheral resistance, rate of sweat secretion rate, and shivering |
Alter heat gains/losses | |||||
Mean arterial pressure | 93 mmHg | Mechanosensors (carotid sinus and aortic arch) | Medulla | Heart and blood vessels | Alter heart rate, peripheral resistance, inotropic state of the heart, and venomotor tone |
Blood volume (effective circulating volume) | 5 liters | Mechanosensors | Medulla | Heart | Alter heart rate, peripheral resistance, and inotropic state of the heart |
(Blood vessels: carotid bodies) | Hypothalamus | Blood vessels | Alter Na+ and water reabsorption | ||
(Heart: atria and ventricle) | Atria | Kidneys | Alter water absorption | ||
(Kidney: juxtaglomerular apparatus and renal afferent arterioles) | Kidney | Intestine | |||
Blood osmolality | 280–296 mosM/kg | Osmosensors (hypothalamus) | Hypothalamus | Kidneys | Alter water reabsorption |
This table includes commonly found components of control systems involved in physiological regulation (i.e., homeostasis). This is not meant to be an exhaustive list but rather reflect the current understanding of homeostatically regulated variables that undergraduate physiology students should understand and be able to apply to problems (e.g., making predictions about responses to perturbations or explaining symptoms of disease).
A potential sticky point occurs when textbooks identify variables as homeostatically regulated even though the system involved does not have all of the required components. The proposition that certain metabolic waste products (e.g., nitrogenous wastes, bilirubin, and creatinine) are homeostatically regulated illustrates such a failure. We are not suggesting that the levels of these substances are not kept relatively constant by steady-state processes in the body. Rather, the concentrations of these substances are not maintained by a system that meets the definition of a homeostatic mechanism listed above. The body does not possess a physiological sensor for detecting these substances in the ECF and therefore cannot homeostatically regulate the ECF concentration of these substances.
Conversely, some mechanisms for controlling the level of a physiological variable include one component of the model (e.g., negative feedback) and may give the appearance of homeostatic regulation but, in the final analysis, do not meet all criteria and should not be considered homeostatic. For example, textbook diagrams illustrating control of blood cortisol levels show several negative feedback loops. This can cause students to think that cortisol is a regulated variable. However, the sensed variable(s) in this system is(are) the variables (e.g., blood glucose or “stress”) whose values are processed by the higher brain centers or hypothalamus and result in the release of corticotropin-releasing hormone. The result of the negative feedback loops involving adrenocorticotropic hormone and cortisol is a modulation of the release rate of the respective hormones. Therefore, corticotropin-releasing hormone, adrenocorticotropic hormone, and cortisol should not be considered homeostatically regulated variables. They are signaling elements controlling the effectors that determine the value of the regulated variable(s).
Another possible source of confusion about the identification of regulated variables arises when a physiological variable is regulated under one set of circumstances but behaves as a controlled variable under other circumstances. This can happen if a regulated variable is under the control of two different homeostatic systems or if a regulated variable can be “coopted” by another homeostatic system. This often happens if a physiological variable plays a role in more than one function in the body.
It is here that the concept of nested homeostasis or hierarchies of homeostats can be helpful. Carpenter () has pointed out that there are circumstances in which the maintenance of one regulated variable at its set point value is more important for continued viability of the organism than the simultaneous regulation of another variable.
One example of this is provided by the value of Pco2 in the ECF. As a variable in the internal environment that affects cell viability, Pco2 meets all of the criteria for a homeostatically regulated variable. Pco2 in the ECF depends on the action of respiratory muscles that alter the rate and depth of ventilation. As such, Pco2 in the ECF is maintained within defined limits by a regulatory system that senses Pco2 and operates by negative feedback. However, as any student of acid-base physiology knows, Pco2 in the ECF is not maintained relatively constant during compensatory adjustments in the acid-base balance of the body. From the perspective of H+ homeostasis, Pco2 functions as a controlled variable.
At this point, some of our students might ask “Which is it? Is Pco2 a regulated variable or is it a controlled variable?” Our answer is that Pco2 is “both,” and we can explain this using the idea of nested homeostatic mechanisms. There are circumstance in which it is more important to maintain arterial H+ concentration (pH) in the normal range that maintaining a constant Pco2, perhaps because of the particular impact of the H+ concentration on cell survival. Therefore, effective regulation of the H+ concentration of the ECF can only be achieved by allowing Pco2 to dramatically vary from its normal range during acid-base disturbances. By introducing the concept of nested homeostatic mechanisms, we have refined how we view Pco2 as a homeostatically regulated variable, and we have offered another way to resolve other, “sticky” situations where the authenticity of a homeostatically regulated variable might be called into question.
Best Practices in Teaching Homeostasis
Given the centrality of the concept of homeostasis (, ), one would expect that both instructional resources and instructors would provide a consistent model of the concept and apply this model to appropriate systems in which variables are sensed and maintained relatively constant.
However, examination of undergraduate textbooks revealed that this is not the case (17). The problems found include, but were not limited to, inconsistent language used to describe the phenomenon and incomplete or inadequate pictorial representations of the model. In addition, texts often define homeostasis early in the narrative but fail to reinforce application of the model when specific regulatory mechanisms are discussed (17).
Furthermore, our work focusing on developing a concept inventory for homeostatic regulation (12, 13) revealed considerable confusion among faculty members regarding the concept. We think this confusion may stem, in part, from the level of faculty uncertainty about the concept and degree of complexity of homeostatic regulatory mechanisms. Our discussion of the sticky points associated with homeostasis is an attempt to suggest potential sources of this confusion and to indicate ways that instructors can work through these difficulties.
How do we ameliorate this situation? We propose five strategies that will help in approaching the problem.
1. Faculty members members should adopt a standard set of terms associated with the model. There is inconsistency within and among textbooks with respect to the names for critical components of the model. We propose the terminology shown in Table 2 to be used when discussing homeostatic regulatory mechanisms.
Table 2.
Term | |
---|---|
Control center (or integrator) | The control center consists of an error detector and controller. It receives signals (information) from sensors, compares information (value of regulated variable) with the set point, integrates information from all sensors, and sends output signals (sends instructions or commands) to increase or decrease the activity of effectors. The control center determines and initiates the appropriate physiological response to any change or disturbance of the internal environment |
Controller | The component of the control center that receives signals (information) from the error detector and sends output signals (instructions or commands) to increase or decrease the activity of effectors. The controller initiates the appropriate physiological response to an error signal resulting from a change or disturbance of the regulated (sensed) variable. |
Effector | A component whose activity or action contributes to determining the value of any variable the system. In this model, the effectors determine the value of the regulated (sensed) variable. |
Error detector | The component in the control center that determines (calculates) the difference between the set point value and the actual value of the regulated (sensed) variable. The error detector generates the error signal that is used to determine the output of the control center. |
Error signal | A signal that represents the difference between the set point value and the actual value of the regulated variable. The error signal is one of the input signals to the controller. |
External environment | The world outside of the body and its “state.” The state or conditions in the outside world can determine the state of many internal properties of the organism. |
Integrator | This is another term for the control center. The integrator processes information from the sensor and those components that determine the set point, determines any error signal present, and sends output signals (instructions or commands) to increase or decrease the activity of effectors. |
Internal environment | The internal environment is the extracellular fluid compartment. This is the environment in which the body's cells live. It is what Bernard meant by the “internal milieu.” |
Homeostasis | The maintenance of a relatively stable internal environment by an organism in the face of a changing external environment and varying internal activity using negative feedback mechanisms to minimize an error signal. |
Negative feedback | A control mechanism where the action of the effector (response) opposes a change in the regulated variable and returns it back toward the set point value. |
Nonregulated variable (controlled variable) | A variable whose value changes in response to effector activity but whose value is not directly sensed by the system. Controlled variables contribute to determination of the regulated variable. For example, heart rate and stroke volume (controlled variables) contribute to determining cardiac output (another controlled variable) that contributes to arterial blood pressure (a regulated variable). |
Perturbation (disturbance) | Any change in the internal or external environment that causes a change to a homeostatically regulated variable. Physiologically induced changes in the set point would not be considered a perturbation. |
Regulated variable (sensed variable) | Any variable for which sensors are present in the system and the value of which is kept within limits by a negative feedback system in the face of perturbations in the system. A regulated variable is any property or condition of the extracellular fluid that is kept relatively constant in the internal environment in order to ensure the viability (survival) of the organism. |
Response | The change in the function or action of an effector. |
Sensor (Receptor) | A “device” that measures the magnitude of some variable by generating an output signal (neural or hormonal) that is proportional to the magnitude of the stimulus. A sensor is a measuring “device.” For some regulated variables, sensors are specialized sensory cells or “sensory receptors,” e.g., thermoreceptors, baroreceptors, or osmoreceptors. For other regulated variables, sensors are cellular components, e.g., the Ca2+-sensing receptor (a G protein-coupled receptor that senses blood Ca2+ in the parathyroid gland). |
Set point | The range of values (range of magnitudes) of the regulated variable that the system attempts to maintain. Set point refers to the “desired value.” The set point is generally not a single value; it is a range of values. |
A glossary of terms used in discussing the core concept of homeostasis. The components of a homeostatically regulated system (Fig. 1) are defined here as are some other terms that occur in teaching this concept.
2. A standard standard pictorial representation of the model should be adopted when initially explaining homeostasis, and it should be used to frame the discussion of the specific system being considered. Figure 1 shows such a diagram.
The argument could be made that this diagram may be difficult for undergraduate students to understand. This may be the rationale for presenting the much-simplified diagrams found in most undergraduate texts (17). However, because these simple diagrams do not explicitly include all components of a homeostatic regulatory system (e.g., a set point), they may be a source of the misconceptions discussed as sticky points. As a result, students may not recognize that an essential feature of homeostatic regulatory systems is minimizing an error signal. A simplified representation of the model that includes the critical components of the regulatory system is shown in Fig. 2. Depending on the course content and level of the student, this model can be expanded to add more levels of complexity as are required.
Simplified representation of a homeostatic regulatory system. Several components shown in Fig. 1 are combined in this representation. The reader should refer to Table 1 to find correspondence between components of physiologically significant homeostatic regulatory systems and this simplified representation. For example, chemosensors in the carotid bodies and aortic body are “sensors,” the brain stem is the “control center,” and the diaphragm and other respiratory muscles are “effectors” in the homeostatic regulatory system for arterial Po2.
3. Faculty members should introduce the concept of homeostatic regulation early in the course and continue to apply and hence reinforce the model as each new homeostatic system is encountered. It is important to continue to use the standard terminology and visual representation as recommended in the first and second points above. Students tend to neither spontaneously or readily generalize their use of core concepts. It is therefore incumbent on the instructor to create a learning environment where this kind of transfer behavior is promoted. Faculty members can facilitate this by providing multiple opportunities for students to test and refine their understanding of the core concept of homeostatic regulation.
One way to reinforce the broad application of the model of homeostasis and help students demonstrate that they understand any particular homeostatic mechanism is to have them ask (and answer) a series of questions about each of homeostatically regulated systems they encounter (see Table 3). In doing so, they demonstrate that they can determine the essential components of the mental model needed to define the homeostatic system. The effort to thoroughly and accurately answer these questions will help students uncover gaps in their understanding and will reveal uncertainties in the resource information that they are using.
Table 3.
Questions students should ask about any homeostatically regulated system
What is the homeostatically regulated variable? Is it a property or condition of the extracellular fluid? |
What and where is the sensor? |
What and where is the control center? |
What and where is the effector(s)? How do they alter their activities so as to produce a response? |
Does the response lead to a change in the regulated variable/stimulus consistent with error signal reduction (negative feedback)? |
4. Faculty members should use care when they select and explain the physiological examples or analogical models they chose to introduce and illustrate homeostasis in the classroom. In particular, instructors should ensure that the representative examples they use do not introduce additional misconceptions into student thinking. This is especially so when thermoregulation may be considered as an example of homeostatic regulation.
An informal survey of physiology textbooks indicated that thermoregulation is almost universally used as an example of a homeostatic mechanism. The most likely reasons for this selection are that 1) there is an everyday, seemingly easy to understand process involving the regulation of air temperature in room or building (i.e., the operation of a furnace and an air conditioner) and 2) the body's physiological responses are commonly and obviously observable and/or experienced by the learner (sweating, shivering, and changes in skin coloration). However, based on our description of the typical homeostatic regulatory system, there are compelling reasons to recommend that caution be taken if thermoregulation is used as the initial and representative example of homeostasis.
But it’s unclear how that logic holds up after closer inspection. Reddit windows 10 piracy.
Most concerning, the typical home heating and cooling system operates in a manner that is distinctly different from mechanisms of human thermoregulation. The effectors in most houses, the furnace and air conditioner, operate in a full-on/full-off manner. For example, when the temperature at the thermostat falls below the value that has been dialed in (the set point temperature), the furnace turns on and stays on at maximum output until the temperature returns to the set point value. However, this is not how the human thermoregulatory system functions or how other homeostatic mechanisms operate. One potential consequence of using this model system to illustrate a homeostatic system is the creation of a common student misconception that homeostatic mechanisms operate in an on/off manner (12, 24), a sticky point we have addressed above. Faculty members need to help students overcome this problem area if they chose to use thermoregulation as a representative example of homeostasis.
What alternatives might be recommended? We suggest the automobile cruise control as a helpful nonbiological analog for homeostasis. The use of cruise control is not an uncommon activity for students, and, as we have described previously, the operation of a cruise control is theoretically easy to understand. What about a physiological example to represent homeostasis? A review of Table 1 would suggest the insulin-mediated system for blood glucose regulation during the fed state has much to recommend it. Students are generally familiar with the particulars of the system from either previous coursework or from personal experience. Other systems are likely to be less accessible to the beginning student of physiology.
However, faculty members should be aware that blood glucose regulation is not without its downsides as a representative example of homeostatic regulation. It is not easy to identify or explain the operation of the glucose sensor, the set point, and the controller involved in glucose homeostasis. Furthermore, there is probably no widely understood analog to glucose regulation that can be easily drawn from everyday life. Neither cruise controls, navigation systems on airplanes, autofocuses on cameras or other common, nor everyday examples of servomechanisms fully correspond to the operation of the feedback system involved in regulating blood glucose during the fed state. This points out the tradeoffs that must be made when any particular example or model is adopted to represent homeostatic regulation. Recognizing this, the use of a physiological control system such as glucose regulation during the fed state, where the effectors operate continuously, seems preferable to thermoregulation as a representative example for teaching the concept of homeostatic regulation.
5. When discussing discussing organismal physiology, restrict the use of the term “homeostatic regulation” to mechanisms related to maintaining consistency of the internal environment (i.e., the ECF).
Adopting these five strategies will provide students with a consistent framework for building their own mental models of specific homeostatic mechanisms and will help them recognize the functional similarities among different homeostatic regulatory systems at the organismal level. Because of its widespread application to different systems in organismal biology, homeostasis is one of the most important unifying ideas in physiology (, ). To construct a robust and enduring understanding of this concept, students need the proper tools. By giving them a precise and consistent terminology and encouraging them to use a standardized pictorial representation of the homeostatic model, we enable them to build a proper foundation for comprehending homeostatic systems. By making students aware of the potential sources of confusion surrounding the concept of homeostasis, i.e., the sticky points, we help prevent their thinking from becoming misguided or out of square. By doing so, we set the stage for our students to develop an accurate understanding of a wide range of physiological phenomena and to arrive at an integrated sense of the “wisdom of the body.”
GRANTS
This work was supported by National Science Foundation Grant DUE-1043443.
DISCLAIMER
Any results, conclusions or recommendations expressed in this article are those of the authors and do not necessarily reflect the views of the NSF.
DISCLOSURES
No conflicts of interest, financial or otherwise, are declared by the author(s).
AUTHOR CONTRIBUTIONS
Author contributions: H.I.M., W.H.C., and J. Michael conception and design of research; H.I.M. and W.H.C. analyzed data; H.I.M., W.H.C., and J. Michael drafted manuscript; H.I.M., W.H.C., J. Michael, J. McFarland, M.P.W., and A.W. edited and revised manuscript; H.I.M., W.H.C., J. Michael, J. McFarland, M.P.W., and A.W. approved final version of manuscript; J. Michael prepared figures.
REFERENCES
1. Association of American Medical Colleges-Howard Hughes Medical Institute Committee. Scientific Foundations for Future Physicians. Report of the AAMC-HHMI Committee. Washington, DC: Association of American Medical Colleges, 2009. [Google Scholar]
2. Baylis LE.Living Control Systems. San Francisco, CA: Freeman and Company, 1966. [Google Scholar]
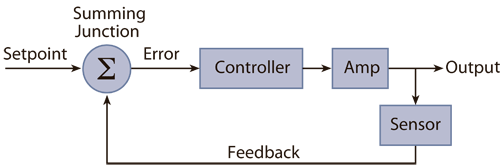
3. Bernard C.Lecons sur les Phenomenes de la vie Communs aux Animaux and aux Vegetaux. Paris: Bailliere, 1878. [Google Scholar]

4. Best CH, Taylor NB.The Physiological Basis of Medical Practice (8th ed). Baltimore, MD: Williams & Wilkins, 1966. [Google Scholar]
5. Brobeck JR.Exchange, control, and regulation. In: Physiological Controls and Regulations, edited by Yamamoto W, Brobeck JR.Philidelphia, PA: Saunders, 1965, pp. 1–13. [Google Scholar]
6. Cannon WB.Organization for physiological homeostasis. Physiol Rev9: 399–431, 1929. [Google Scholar]
7. Carpenter RH.Homeostasis: a plea for a unified approach. Adv Physiol Educ28: 180–187, 2004. [PubMed] [Google Scholar]
8. Cooper SJ.From Claude Bernard to Walter Cannon. Emergence of the concept of homeostasis. Appetite51: 419–427, 2008. [PubMed] [Google Scholar]
9. Fulton JF. (editor). A Textbook of Physiology (16th ed). Philadelphia, PA: Saunders, 1949. [Google Scholar]
Positive Feedback Loop
10. Guyton A.Textbook of Medical Physiology (3rd ed). Philadelphia, PA: Saunders, 1966. [Google Scholar]
11. Houssay BA.Human Physiology (2nd ed). New York: McGraw-Hill, 1955. [Google Scholar]
12. McFarland J, Michael J, Wenderoth MP, Modell H, Wright A, Cliff W.Conceptual frameworks and misconceptions associated with core principles of physiology, including homeostasis. FASEB J26: 720.4, 2012. [Google Scholar]
13. McFarland J, Michael J, Wenderoth MP, Modell H, Wright A, Cliff W.Conceptual assessment of physiology: development of a concept inventory for homeostasis. FASEB J28: 531.13, 2014. [Google Scholar]
14. Michael J.Conceptual assessment in the biological sciences: a National Science Foundation-sponsored workshop. Adv Physiol Educ31: 389–391, 2007. [PubMed] [Google Scholar]
15. Michael J, Modell H, McFarland J, Cliff W.The “core principles” of physiology: what should students understand?Adv Physiol Educ33: 10–16, 2009. [PubMed] [Google Scholar]
16. Michael J, McFarland J.The core principles (“big ideas”) of physiology: results of faculty surveys. Adv Physiol Educ35: 336–341, 2011. [PubMed] [Google Scholar]
17. Michael J, McFarland J, Cliff W, Modell H, Wenderoth MP, Wright A.Homeostasis in undergraduate physiology textbooks. FASEB J27: 739.4, 2013. [Google Scholar]
18. Milhorn HT.The Application of Control Theory to Physiological Systems. Philadelphia, PA: Saunders, 1966. [Google Scholar]
19. Milsum JH.Biological Control Systems Analysis. New York: McGraw-Hill, 1966. [Google Scholar]
20. Riggs DS.Control Theory and Physiological Feedback Mechanisms. Baltimore: Williams & Wilkins, 1970. [Google Scholar]
21. Vander A, Sherman JH, Luciano DS.Human Physiology: the Mechanisms of Body Function (8th ed). New York: McGraw-Hill, 2001. [Google Scholar]
Homeostasis Effector
22. Woods HA, Wilson JK.An information hypothesis for the evolution of homeostasis. Trends Ecol Evol28: 283–289, 2013. [PubMed] [Google Scholar]
23. Woods SC, Ramsey DS.Homeostasis: beyond Curt Richter. Appetite49: 388–398, 2007. [PMC free article] [PubMed] [Google Scholar]
24. Wright A, McFarland J, Cliff W, Michael J, Modell H, Wenderoth MP.Preliminary results on the prevalence of physiology students' homeostatic misconceptions. FASEB J27: 739.5, 2013. [Google Scholar]
Articles from Advances in Physiology Education are provided here courtesy of American Physiological Society